Introduction
A Van de Graaff generator, is an electrostatic generator which uses a moving belt to accumulate very high amounts of electrical potential on a hollow metal globe on the top of the stand. It was invented by American physicist Robert J. Van de Graaff in 1929. The potential difference achieved in modern Van de Graaff generators can reach 5 megavolts. A tabletop version can produce on the order of 100,000 volts and can store enough energy to produce a visible spark. A Van de Graaff generator operates by transferring electric charge from a moving belt to a terminal. The high voltages generated by the Van de Graaff generator can be used for accelerating subatomic particles to high speeds, making the generator a useful tool for fundamental physics research. it is also used for accelerating electrons to sterilize food and process materials, accelerating protons for nuclear physics experiments, driving X-ray tubes, etc.
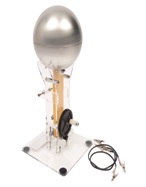
THEORY
Working of the generator is based on two principles:
- Discharging action of sharp points, ie., electric discharge takes place in air or gases readily, at pointed conductors.
- If the charged conductor is brought in to internal contact with a hollow conductor, all of its charge transfers to the surface of the hollow conductor no matter how high the potential of the latter may be.
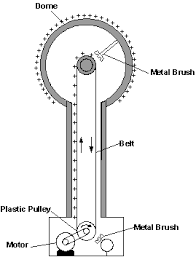
working
A simple Van de Graaff-generator consists of a belt of silk,or a similar flexible dielectric material, running over two metal pulleys, one of which is surrounded by a hollow metal sphere. Two electrodes, (2) and (7), in the form of comb-shaped rows of sharp metal points, are positioned respectively near to the bottom of the lower pulley and inside the sphere, over the upper pulley. Comb (2) is connected to the sphere, and comb (7) to the ground. A high DC potential (with respect to earth) is applied to roller (3); a positive potential in this example.
As the belt passes in front of the lower comb, it receives negative charge that escapes from its points due to the influence of the electric field around the lower pulley, which ionizes the air at the points. As the belt touches the lower roller (6), it transfers some electrons, leaving the roller with a negative charge (if it is insulated from the terminal), which added to the negative charge in the belt generates enough electric field to ionize the air at the points of the upper comb. Electrons then leak from the belt to the upper comb and to the terminal, leaving the belt positively charged as it returns down and the terminal negatively charged.
The sphere shields the upper roller and comb from the electric field generated by charges that accumulate at the outer surface of it, causing the discharge and change of polarity of the belt at the upper roller to occur practically as if the terminal were grounded. As the belt continues to move, a constant ‘charging current’ travels via the belt, and the sphere continues to accumulate negative charge until the rate that charge is being lost (through leakage and corona discharges) equals the charging current. The larger the sphere and the farther it is from ground, the higher will be its final potential.
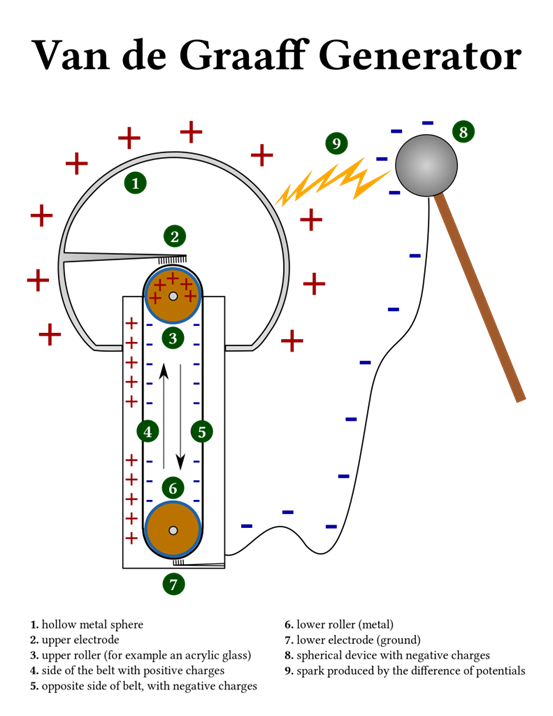
Another method for building Van de Graaff generators is to use the triboelectric effect. The friction between the belt and the rollers, one of them now made of insulating material, or both made with insulating materials at different positions on the triboelectric scale, one above and other below the material of the belt, charges the rollers with opposite polarities. The strong e-field from the rollers then induces a corona discharge at the tips of the pointed comb electrodes. The electrodes then “spray” a charge onto the belt which is opposite in polarity to the charge on the rollers.
The remaining operation is otherwise the same as the voltage-injecting version above. This type of generator is easier to build for science fair or homemade projects, since it does not require a potentially dangerous high-voltage source. The trade-off is that it cannot build up as high a voltage as the other type, that cannot also be easily regulated, and operation may become difficult under humid conditions (which can severely reduce triboelectric effects). Finally, since the position of the rollers can be reversed, the accumulated charge on the hollow metal sphere can either be positive or negative.
A Van de Graaff generator terminal does not need to be sphere-shaped to work, and in fact, the optimum shape is a sphere with an inward curve around the hole where the belt enters. Since electrically charged conductors have no e-field inside, charges can be added continuously. A rounded terminal minimizes the electric field around it, allowing greater potentials to be achieved without ionization of the surrounding air, or other dielectric gas. Outside the sphere, the e-field quickly becomes very strong and applying charges from the outside would soon be prevented by the field. Since a Van de Graaff generator can supply the same small current at almost any level of electrical potential, it is an example of a nearly ideal current source. The maximum achievable potential is approximately equal to the sphere’s radius multiplied by the e-field where corona discharges begin to form within the surrounding gas. For example, a polished spherical electrode 30 cm in diameter immersed in air at STP (which has a breakdown voltage of about 30 kV/cm) could be expected to develop a maximum voltage of about 450 kV.
EXPERIMENTAL PROCEDURE AND CALCULATIONS
If we have a large conducting spherical shell of radius ‘R’ on which we place a charge Q, it spreads itself uniformly all over the sphere. The field outside the sphere is just that of a point charge Q at the centre, while the field inside the sphere vanishes.
So the potential outside is that of point charge and inside it is constant. The potential inside the conducting
sphere =
Now suppose that we introduce a small sphere of radius ‘r’, carrying a charge q, into the large one and place it at the centre. The potential due to this new charge has following values.
Potential due to small sphere of radius r carrying charge
Potential at the surface of large shell of radius R
Taking both charges q and Q in to account we have for the total potential V and the potential difference given by,
Now assume that q is positive. We see that, independent of the amount of charge Q that may have accumulated on the larger sphere,
it is always at a higher potential: the difference V(r) – V(R) is positive.
The potential due to Q is constant up to radius R and so cancels out in the difference. This means that if we connect the smaller and larger sphere by a wire, the charge q on the former will immediately flow on to the matter, even though the charge Q may be quite large. The natural tendency is for positive charge to move higher to lower potential. Thus, provided we are somehow able to introduce the small charged sphere into the larger one, we can in this way pile up larger and larger amount of charge on the latter. The potential of the outer sphere would also keep rising, at least until it reaches the breakdown field of air.
OBSERVATIONS
It consists of a large metal sphere mounted on high insulating supports. An endless belt b, made of insulating material such as rubber, passes over the vertical pulleys P1 and P2. The pulley P2 is at the centre of the metal sphere and the pulley P1 is vertically below P2.
The belt is run by an electric motor M. B1and B2 are two metal brushes called collecting combs. The positive terminal of a high tension source (HT) is connected to the comb B1.
Due to the process called action of points, charges are accumulated at the pointed ends of the comb, the field increases and ionizes the air near them. The positive charges in air are repelled and get deposited on the belt due to corona discharge. The charges are carried by the belt upwards as it moves. When the positively charged proton of the belt comes in front of the brush B2, by the same process of action of points and corona discharge occurs and the metal sphere acquires positive charges.
The positive charges are uniformly distributed over the surface of the sphere. Due to the action of points by the negative charges carried by the gas in front of the comb B2, the positive charge of the belt is neutralized. The uncharged portion of the belt returns down collects the positive charge from B1 which in turn is collected by B2. The charge transfer process is repeated.
As more and more positive charges are imparted to the sphere, its positive potential goes on rising until a surface maximum is reached. If the potential goes beyond this, insulation property of air breaks down and the sphere gets discharged. The breakdown of air takes place in an enclosed steel chamber filled with nitrogen at high pressure.
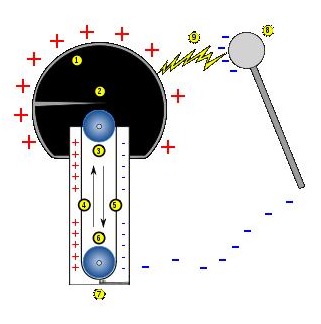
CONCLUSIONS
The Van de Graaff generator in its simplest form is seen as a didactical instrument, because of its solidity and simple construction. This generator became an ideal demonstration apparatus of electrostatic influence machine. Van de Graaff high voltage electrostatic generators are used in public schools and universities for teaching basic principles of high voltage electrostatics charge and laws of electrostatics.
The Van de Graaff generator is extremely useful because it produces electric fields which are strong enough to be measured, manipulated, felt directly and played with. Men have always been fascinated by lightning and big sparks, therefore this machine was always the ideal and much appreciated display in important exhibitions, science museums and science centers. Table top Van de Graaff generators develop over 200,000 Volts and floor models offer up to 1,000,000 Volts of high voltage lightning electrical discharges.